Νόμπελ Φυσικής 2023
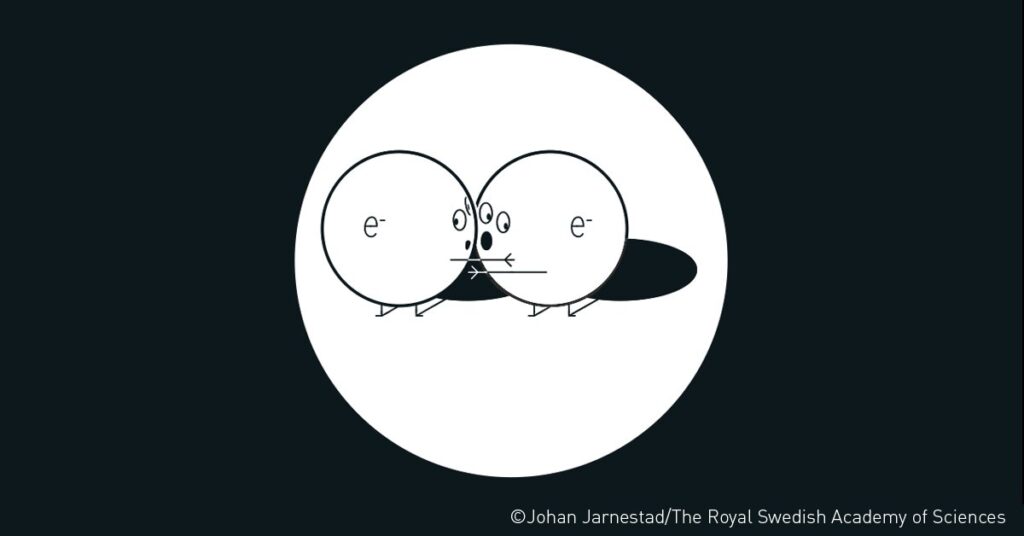
Οχι σπάνια, η Βασιλική Σουηδική Ακαδημία Επιστημών απονέμει το ετήσιο βραβείο Νομπέλ Φυσικής σε επιστήμονες που συνέβαλαν στην εξιχνίαση θεμελιωδών μυστηρίων της ύλης και του σύμπαντος, στις κλίμακες του πολύ μικρού και του πολύ μεγάλου – από τα εξωτικά στοιχειώδη σωματίδια του κβαντικού μικρόκοσμου μέχρι τις μαύρες τρύπες και τη Μεγάλη Εκρηξη. Αλλοτε, πάλι, επιβραβεύει ερευνητές που ασχολούνται με πιο γήινα προβλήματα, με άμεσο αντίκτυπο στη ζωή μας, όπως συνέβη το 2018, όταν τρεις επιστήμονες τιμήθηκαν για τις ανακαλύψεις τους στο πεδίο των λέιζερ, ή το 2021, όταν ισάριθμοι συνάδελφοί τους έλαβαν το βραβείο για τη συμβολή τους στην κατανόηση πολύπλοκων συστημάτων, όπως το παγκόσμιο κλίμα.
Με τη χθεσινή απόφασή της, η Σουηδική Ακαδημία στάθηκε στη γραμμή συνάντησης των δύο κόσμων. Το βραβείο Νομπέλ Φυσικής του 2023 θα μοιραστούν ο Γάλλος Πιερ Αγκοστινί, ο Ούγγρος Φέρεντς Κράουτς και η Γαλλίδα Αν Λ’Ουιγιέ για τις έρευνές τους σχετικά με τις ταχύτατες αλλαγές που υφίστανται τα ηλεκτρόνια μέσα στα άτομα και τα μόρια, στους δομικούς λίθους του κόσμου που μας περιβάλλει.
Οι ανακαλύψεις τους άνοιξαν νέους δρόμους στη βασική έρευνα, ενώ ταυτόχρονα δημιούργησαν ελπίδες για καινοτόμους εφαρμογές σε πεδία όπως η επινόηση νέων ηλεκτρονικών διατάξεων, ακριβέστερων μεθόδων ιατρικής διάγνωσης και παραγωγής καινούργιων χημικών ενώσεων.
Τα ηλεκτρόνια αντιπροσωπεύουν, τυπικά, μόλις το 1/3.600 της μάζας ενός μορίου (καθώς είναι κατά πολύ ελαφρύτερα από τα νετρονίων και τα πρωτόνια που τα συναποτελούν), αλλά οι αλλαγές στην κατάστασή τους παράγουν το σύνολο των φυσικών και χημικών φαινομένων που εξελίσσονται στον κόσμο γύρω μας, με εξαίρεση εκείνα που λαμβάνουν χώρα στους πυρηνικούς αντιδραστήρες, στους επιταχυντές σωματιδίων ή στα αστέρια. Οι μεταβολές στην κίνηση ή στην ενέργειά τους είναι τόσο ταχείες, που οι επιστήμονες πίστευαν μέχρι πρότινος ότι δεν θα ήταν δυνατόν να τις αιχμαλωτίσουν με οποιαδήποτε απεικονιστική τεχνική.
Ενδεικτικά, για να φωτογραφίσει κάποιος έναν άνθρωπο που βαδίζει στον δρόμο θα πρέπει το κλείστρο της φωτογραφικής μηχανής να λειτουργήσει με ταχύτητα 1/250 του δευτερολέπτου (sec). Για ένα κινούμενο αυτοκίνητο, η απαιτούμενη ταχύτητα γίνεται 1/4.000 sec. Αλλά οι ταχύτητες μεταβολής των ηλεκτρονίων είναι ασύλληπτα γρήγορες, αφού εξελίσσονται μέσα σε 1 attosecond, δηλαδή ένα πεντάκις εκατομμυριοστό του δευτερολέπτου. Για να πάρουμε μια ιδέα, είναι το απειροστό διάστημα που προκύπτει αν διαιρέσουμε ένα δευτερόλεπτο διά 1.000 έξι φορές.
Η μέθοδος
Αυτό το άλυτο μέχρι χθες πρόβλημα κατάφεραν να αντιμετωπίσουν οι τρεις επιστήμονες, δημιουργώντας βραχύτατους φωτεινούς παλμούς λέιζερ της τάξης των attosecond και χρησιμοποιώντας τους στη συνέχεια για να διακρίνουν τις αλλαγές θέσης ή ενέργειας που υφίστανται τα ηλεκτρόνια μέσα στα άτομα ή στα μόρια. Ο Κράουτς και η ομάδα του ήταν οι πρώτοι που παρήγαγαν τέτοιους παλμούς στην αρχή της δεκαετίας του 2000, ενώ κάτι ανάλογο έγινε με την ομάδα του Αγκοστινί.
Με χρήση βραχέων παλμών λέιζερ, κατάφεραν να απεικονίσουν τις ταχύτατες αλλαγές των ηλεκτρονίων στα άτομα και στα μόρια.
Και οι δύο ομάδες στηρίχτηκαν σε προηγούμενες έρευνες της Λ’Ουιγιέ, από τη δεκαετία του ’80, όταν η Γαλλίδα φυσικός ανακάλυψε ένα νέο φαινόμενο στην αλληλεπίδραση ακτινοβολίας λέιζερ με άτομα αερίου, το οποίο άνοιξε τον δρόμο για τη δημιουργία των ταχύτατων φωτεινών παλμών.
Ωστόσο, όπως εξηγεί η Γαλλίδα επιστήμονας, ακόμη και με τις πρωτοποριακές μεθόδους των τριών επιστημόνων, κανείς δεν μπορεί πραγματικά να «δει» ένα ηλεκτρόνιο, αφού, όπως προβλέπει η κβαντική φυσική, δεν συμπεριφέρεται ως κλασικό σωματίδιο. «Η εικόνα είναι πολύ θολή. Μπορείς να δεις αν το ηλεκτρόνιο βρίσκεται από τη μια ή την άλλη πλευρά του μορίου, όχι όμως πολύ περισσότερα πράγματα. Τα ηλεκτρόνια μοιάζουν πιο πολύ με κύματα και αυτό που μπορούμε να μετρήσουμε με τις διατάξεις είναι η κορυφογραμμή αυτών των κυμάτων», διευκρινίζει.
Εστω κι έτσι, οι ανακαλύψεις των τριών επιστημόνων έχουν ήδη ανοίξει δρόμους για καινούργιες εφαρμογές, όπως η αποτελεσματικότερη ανίχνευση παθογόνων ουσιών μέσα σε δείγματα αίματος.
Από το 1901, οπότε άρχισε η απονομή των βραβείων Νομπέλ Φυσικής, έχουν πραγματοποιηθεί 117 απονομές και έχουν τιμηθεί με το εν λόγω βραβείο 225 επιστήμονες, συμπεριλαμβανομένων ιερών τεράτων της επιστήμης όπως ο Αλμπερτ Αϊνστάιν, ο Μαξ Πλανκ, ο Νιλς Μπορ, ο Ερβιν Σρέντινγκερ, ο Βέρνερ Χάιζενμπεργκ και τόσοι άλλοι. Ανάμεσά τους διακρίνονταν μέχρι χθες μόνο τέσσερις γυναίκες, με πρώτη τη Μαρί Κιουρί, που μοιράστηκε με τον σύζυγό της Πιερ και άλλους τρεις ερευνητές το βραβείο του 1903 για την ανακάλυψη της ραδιενέργειας.
Ποιοι είναι
Χθες, η Αν Λ’Ουιγιέ έγινε η πέμπτη γυναίκα που γνωρίζει την ύψιστη τιμητική διάκριση. Καθηγήτρια στο Πανεπιστήμιο Λουντ της Σουηδίας, πληροφορήθηκε τη βράβευσή της ενώ έδινε διάλεξη στους φοιτητές και στις φοιτήτριές της, αλλά επέμεινε να ολοκληρώσει την παρουσίαση, αν και, όπως αναγνώρισε, «η επόμενη μισή ώρα ήταν δύσκολη». Δεν παρέλειψε, δε, να προσθέσει: «Είναι εκπληκτικό. Οπως γνωρίζετε, δεν υπάρχουν πολλές γυναίκες που να έχουν λάβει το βραβείο, και αυτό το κάνει ξεχωριστό».
Ο Πιερ Αγκοστινί είναι καθηγητής στο Πολιτειακό Πανεπιστήμιο του Οχάιο, στις ΗΠΑ, ενώ ο Φέρεντς Κράουτς εργάζεται ως διευθυντής του Ινστιτούτου Μαξ Πλανκ για την κβαντική οπτική στη Γερμανία. Είναι ο δεύτερος Ούγγρος που τιμάται φέτος με βραβείο Νομπέλ, ύστερα από τη βιοχημικό Καταλίν Κάρικο, η οποία μοιράστηκε με τον Αμερικανό Ντρου Ουάισμαν το Νομπέλ Ιατρικής.
Οι αριθμοί
117 είναι τα Νοµπέλ Φυσικής που έχουν δοθεί από το 1901 έως το 2023.
225 οι βραβευθέντες µε Νοµπέλ Φυσικής.
5 γυναίκες μόνο έχουν τιμηθεί με το βραβείο.
25 ετών ήταν, το 1915, ο νεότερος βραβευθείς, ο Γουίλιαμ Λόρενς Μπραγκ.
96 ετών ήταν, το 2018, ο Αρθουρ Ασκιν, ο γηραιότερος βραβευθείς.
The 2023 Nobel Prize laureates in physics are being recognised for their experiments, which have given humanity new tools for exploring the world of electrons inside atoms and molecules. Pierre Agostini, Ferenc Krausz and Anne L’Huillier have demonstrated a way to create extremely short pulses of light that can be used to measure the rapid processes in which electrons move or change energy.
The laureates’ contributions have enabled the investigation of processes that are so rapid they were previously impossible to follow.
There are potential applications in many different areas. In electronics, for example, it is important to understand and control how electrons behave in a material. Attosecond pulses can also be used to identify different molecules, such as in medical diagnostics.
Through their experiments, this year’s laureates have created flashes of light that are short enough to take snapshots of electrons’ extremely rapid movements. Anne L’Huillier discovered a new effect from laser light’s interaction with atoms in a gas. Pierre Agostini and Ferenc Krausz demonstrated that this effect can be used to create shorter pulses of light than were previously possible.
Electrons in pulses of light
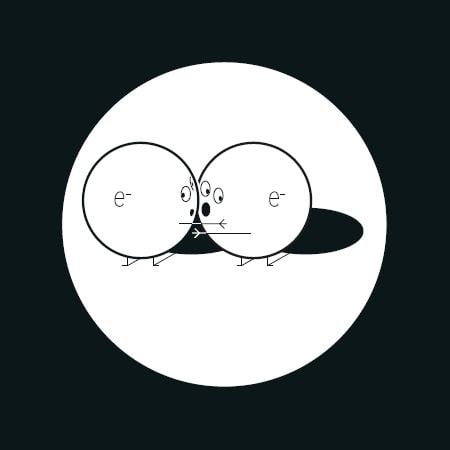
A tiny hummingbird can beat its wings 80 times per second. We are only able to perceive this as a whirring sound and blurred movement. For the human senses, rapid movements blur together, and extremely short events are impossible to observe. We need to use echnological tricks to capture or depict these very brief instants.
High-speed photography and strobe lighting make it possible to capture detailed images of fleeting phenomena. A highly focused photograph of a hummingbird in flight requires an exposure time that is much shorter than a single wingbeat. The faster the event, the faster the picture needs to be taken if it is to capture the instant.
The same principle applies to all the methods used to measure or depict rapid processes; any measurement must be done more quickly than the time it takes for the system being studied to undergo a noticeable change, otherwise the result is vague. This year’s laureates have conducted experiments that demonstrate a method for producing pulses of light that are brief enough to capture images of processes inside atoms and molecules.
Atoms’ natural time scale is incredibly short. In a molecule, atoms can move and turn in millionths of a billionth of a second, femtoseconds. These movements can be studied with the very shortest pulses that can be produced with a laser – but when entire atoms move the timescale is determined by their large and heavy nuclei, which are extremely slow compared to light and nimble electrons. When electrons move inside atoms or molecules, they do it so quickly that changes are blurred out in a femtosecond. In the world of electrons, positions and energies change at speeds of between one and a few hundred attoseconds, where an attosecond is one billionth of a billionth of a second.
An attosecond is so short that that the number of them in one second is the same as the number of seconds that have elapsed since the universe came into existence, 13.8 billion years ago. On a more relatable scale, we can imagine a flash of light being sent from one end of a room to the opposite wall – this takes ten billion attoseconds.
A femtosecond was long regarded as the limit for the flashes of light it was possible to produce. Improving existing technology was not enough to see processes occurring on the amazingly brief timescales of electrons; something entirely new was required. This year’s laureates conducted experiments that opened up the new research field of attosecond physics.
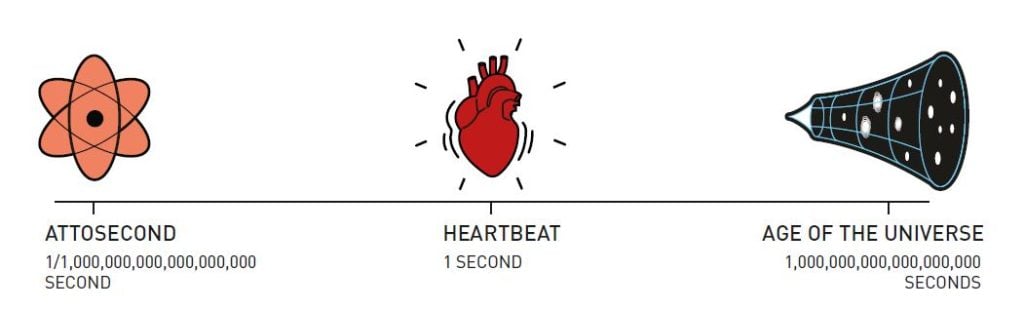
Shorter pulses with the help of high overtones
Light consists of waves – vibrations in electrical and magnetic fields – that move through a vacuum faster than anything else. These have different wavelengths, equivalent to different colours. For example, red light has a wavelength of about 700 nanometres, one hundredth the width of a hair, and it cycles at about four hundred and thirty thousand billion times per second. We can think of the shortest possible pulse of light as the length of a single period in the light wave, the cycle where it swings up to a peak, down to a trough, and back to its starting point. In this case, the wavelengths used in ordinary laser systems are never able to get below a femtosecond, so in the 1980s this was regarded as a hard limit for the shortest possible bursts of light.
The mathematics that describes waves demonstrates that any wave form can be built if enough waves of the right sizes, wavelengths and amplitudes (distances between peaks and troughs) are used. The trick to attosecond pulses is that it is possible to make shorter pulses by combining more and shorter wavelengths.
Observing electrons’ movements on an atomic scale requires short-enough pulses of light, which means combining short waves of many different wavelengths.
To add new wavelengths to light, more than just a laser is necessary; the key to accessing the briefest instant ever studied is a phenomenon that arises when laser light passes through a gas. The light interacts with its atoms and causes overtones – waves that complete a number of entire cycles for each cycle in the original wave. We can compare this to the overtones that give a sound its particular character, allowing us to hear the difference between the same note played on a guitar and a piano.
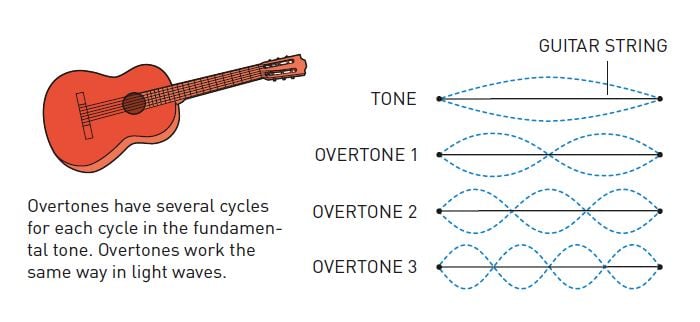
In 1987, Anne L’Huillier and her colleagues at a French laboratory were able to produce and demonstrate overtones using an infrared laser beam that was transmitted through a noble gas. The infrared light caused more and stronger overtones than the laser with shorter wavelengths that had been used in previous experiments. In this experiment, many overtones of about the same light intensity were observed.
In a series of articles, L’Huillier continued to explore this effect during the 1990s, including at her new base, Lund University. Her results contributed to the theoretical understanding of this phenomenon, laying the foundation of the next experimental breakthrough.
Escaping electrons create overtones
When the laser light enters the gas and affects its atoms, it causes electromagnetic vibrations that distort the electric field holding the electrons around the atomic nucleus. The electrons can then escape from the atoms. However, the light’s electrical field vibrates continuously and, when it changes direction, a loose electron may rush back to its atom’s nucleus. During the electron’s
excursion it collected lots of extra energy from the laser light’s electrical field and, to reattach to the nucleus, it must release its excess energy as a pulse of light. These light pulses from the electrons are what create the overtones that appear in the experiments.
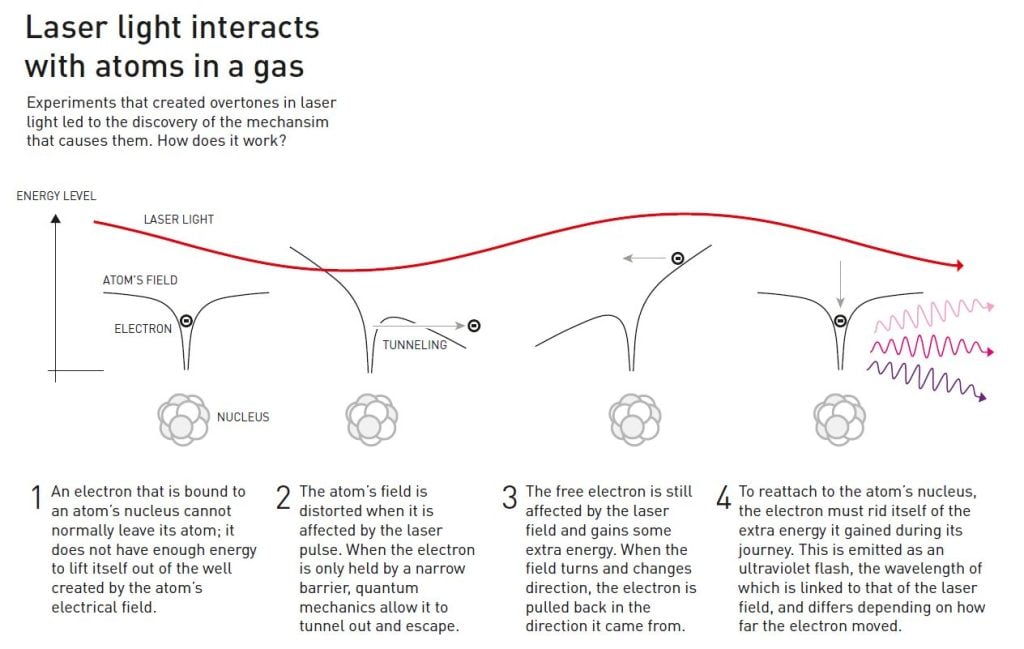
Light’s energy is associated with its wavelength. The energy in the emitted overtones is equivalent to ultraviolet light, which has shorter wavelengths than the light visible to the human eye. Because the energy comes from the laser light’s vibrations, the overtones’ vibration will be elegantly proportional to the wavelength of the original laser pulse. The result of the light’s interaction with many different
atoms is different light waves with a set of specific wavelengths.
Once these overtones exist, they interact with each other. The light becomes more intense when the lightwaves’ peaks coincide, but becomes less intense when the peak in one cycle coincides with the trough of another. In the right circumstances, the overtones coincide so that a series of pulses of ultraviolet light occur, where each pulse is a few hundred attoseconds long. Physicists understood the theory behind this in the 1990s, but the breakthrough in actually identifying and testing the pulses occurred in 2001.
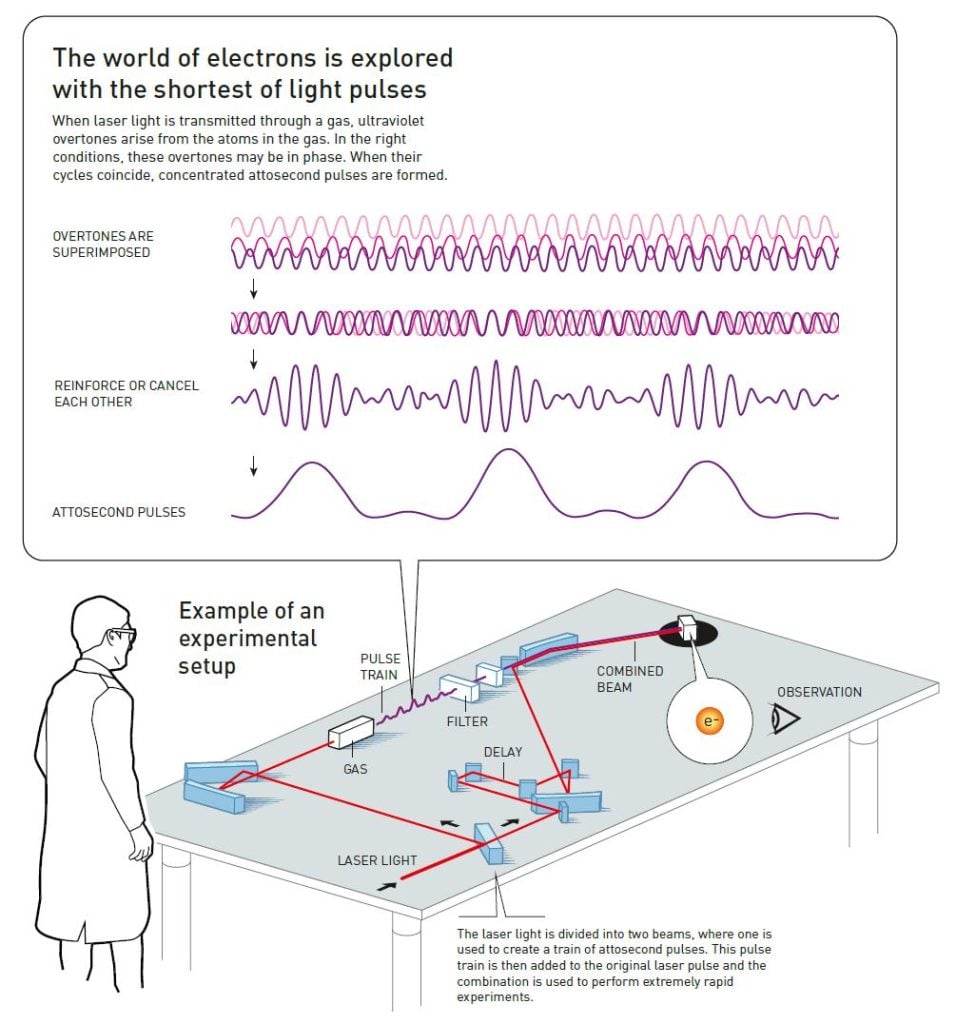
Pierre Agostini and his research group in France succeeded in producing and investigating a series of consecutive light pulses, like a train with carriages. They used a special trick, putting the “pulse train” together with a delayed part of the original laser pulse, to see how the overtones were in phase with each other. This procedure also gave them a measurement for the duration of the pulses
in the train, and they could see that each pulse lasted just 250 attoseconds.
At the same time, Ferenc Krausz and his research group in Austria were working on a technique that could select a single pulse – like a carriage being uncoupled from a train and switched to another track. The pulse they succeeded in isolating lasted 650 attoseconds and the group used it to track and study a process in which electrons were pulled away from their atoms.
These experiments demonstrated that attosecond pulses could be observed and measured, and that they could also be used in new experiments.
Now that the attosecond world has become accessible, these short bursts of light can be used to study the movements of electrons. It is now possible to produce pulses down to just a few dozen attoseconds, and this technology is developing all the time.
Electrons’ movements have become accessible
Attosecond pulses make it possible to measure the time it takes for an electron to be tugged away from an atom, and to examine how the time this takes depends on how tightly the electron is bound to the atom’s nucleus. It is possible to reconstruct how the distribution of electrons oscillates from side to side or place to place in molecules and materials; previously their position could only be measured as an average.
Attosecond pulses can be used to test the internal processes of matter, and to identify different events. These pulses have been used to explore the detailed physics of atoms and molecules, and they have potential applications in areas from electronics to medicine.
For example, attosecond pulses can be used to push molecules, which emit a measurable signal. The signal from the molecules has a special structure, a type of fingerprint that reveals what molecule it is, and the possible applications of this include medical diagnostics.
Further reading
Additional information on this year’s prizes, including a scientific background in English, is available on the website of the Royal Swedish Academy of Sciences, www.kva.se, and at www.nobelprize.org, where you can watch video from the press conferences, the Nobel Lectures and more. Information on exhibitions and activities related to the Nobel Prizes and the Prize in Economic Sciences is available at www.nobelprizemuseum.se.
The Royal Swedish Academy of Sciences has decided to award the Nobel Prize in Physics 2023 to
PIERRE AGOSTINI
PhD 1968 from Aix-Marseille University,
France. Professor at The Ohio State
University, Columbus, USA.
FERENC KRAUSZ
Born 1962 in Mór, Hungary. PhD 1991
from Vienna University of Technology,
Austria. Director at Max Planck Institute
of Quantum Optics, Garching and
Professor at Ludwig-Maximilians-
Universität München, Germany.
ANNE L’HUILLIER
Born 1958 in Paris, France. PhD 1986
from University Pierre and Marie
Curie, Paris, France. Professor at
Lund University, Sweden.
“for experimental methods that generate attosecond pulses of light for the study of electron dynamics in matter”
Σχόλια
Δημοσίευση σχολίου